FIRE RESISTANCE OF REINFORCED CONCRETE AND STEEL STRUCTURES
Keywords:
thermophysical characteristics, fire protective coatings, fireproof building structures, fire protection ability of coatings, fire temperature regimesSynopsis
The scientific bases of ensuring fire resistance of reinforced concrete and steel building structures in the conditions of modern extreme influences are laid.
The current state of fire safety of buildings and structures, as well as approaches, methods and tools for its assessment are analyzed. Analysis of emergencies and fires in the world has shown that the vast majority of them occur in buildings and structures. It is shown that the cause of catastrophic consequences and destruction is the non-compliance of the actual limit of fire resistance of building structures with regulatory requirements. This is due to the imperfection of methods and means of assessing the fire resistance of building structures, including fire-retardant.
To overcome the shortcomings identified during the analysis, the paper develops physical and mathematical models of thermal processes occurring in the fire-retardant reinforced concrete structure. Based on the proposed models, a computational-experimental method for estimating the fire resistance of such structures has been developed. The efficiency of the proposed method was tested by identifying the relationship between the parameters of the fire-retardant plaster coating “Neospray” and the fire resistance of fire-retardant multi-hollow reinforced concrete floor.
The study of fire resistance of steel structures is proposed to be carried out using reduced samples in the form of steel plates with dimensions of 500×500×5 mm. Based on the proposed models, a calculation and experimental method for estimating the fire resistance of steel structures, as well as an algorithm and procedures for its implementation have been developed. The verification of the efficiency of the proposed method was carried out in the ANSYS software package using the aged coating “Phoenix STS” and the coating “Amotherm Steel Wb” under heating conditions at the temperature of the hydrocarbon fire.
The reliability of the developed models and methods is checked. It is established that random errors in temperature measurement significantly affect the accuracy of determining the thermophysical characteristics and limits of fire resistance. In general, the efficiency of the proposed calculation and experimental methods with sufficient accuracy for engineering calculations is confirmed.
Chapters
References
Serant, A. Y., Sokha, Yu. I. (2012). Peculiarities and principles of formation of the systemof public administration of natural and mancaused safety. Efficacy public administration, 32, 457–465.
Pospelov, B., Rybka, E., Togobytska, V., Meleshchenko, R., Danchenko, Y., Butenko, T. et. al. (2019). Construction of the method for semiadaptive threshold scaling transformation when computing recurrent plots. Eastern-European Journal of Enterprise Technologies, 4 (10 (100)), 22–29. doi: http://doi.org/10.15587/17294061.2019.176579
Tiutiunyk, V. V., Ivanets, H. V., Tolkunov, I. A., Stetsyuk, E. I. (2018). System approach for readiness assessment units of civil defense to actions at emergency situations. Scientific Bulletin of National Mining University, 1, 99–105. doi: http://doi.org/10.29202/nvngu/20181/7
Pospelov, B., Rybka, E., Meleshchenko, R., Gornostal, S., Shcherbak, S. (2017). Results of experimental research into correlations between hazardous factors of ignition of materials in premises. Eastern-European Journal of Enterprise Technologies, 6 (10 (90)), 50–56. doi: http://doi.org/10.15587/17294061.2017.117789
Pospelov, B., Rybka, E., Meleshchenko, R., Borodych, P., Gornostal, S. (2019). Development of the method for rapid detection of hazardous atmospheric pollution of cities with the help of recurrence measures. Eastern-European Journal of Enterprise Technologies, 1 (10 (97)), 29–35. doi: http://doi.org/10.15587/17294061.2019.155027
Pospelov, B., Andronov, V., Rybka, E., Krainiukov, O., Karpets, K., Pirohov, O. et. al. (2019). Development of the correlation method for operative detection of recurrent states. Eastern-European Journal of Enterprise Technologies, 6 (4 (102)), 39–46. doi: http://doi.org/10.15587/17294061.2019.187252
Kustov, M., Kalugin, V., Tutunik, V., Tarakhno, O. (2019). Physicochemical principles of the technology of modified pyrotechnic compositions to reduce the chemical pollution of the atmosphere. Voprosy Khimii i Khimicheskoi Tekhnologii, 1, 92–99. doi: http://doi.org/10.32434/03214095201912219299
Vasyukov, A., Loboichenko, V., Bushtec, S. (2016). Identification of bottled natural waters by using direct conductometry. Ecology, Environment and Conservation, 22 (3), 1171–1176.
Dadashov, I., Loboichenko, V., Kireev, A. (2018). Analysis of the ecological characteristics of environment friendly fire fighting chemicals used in extinguishing oil products. Pollution Research Paper, 37 (1), 63–77.
Migalenko, K., Nuianzin, V., Zemlianskyi, A., Dominik, A., Pozdieiev, S. (2018). Development of the technique for restricting the propagation of fire in natural peat ecosystems. Eastern-European Journal of Enterprise Technologies, 1 (10 (91)), 31–37. doi: http://doi.org/10.15587/17294061.2018.121727
Popov, O., Іatsyshyn, A., Kovach, V., Artemchuk, V., Taraduda, D., Sobyna, V. et. al. (2019). Analysis of Possible Causes of NPP Emergencies to Minimize Risk of Their Occurrence. Nuclear and Radiation Safety, 1 (81), 75–80. doi: http://doi.org/10.32918/nrs.2019.1(81).13
Vambol, S., Vambol, V., Kondratenko, O., Suchikova, Y., Hurenko, O. (2017). Assessment of improvement of ecological safety of power plants by arranging the system of pollutant neutralization. Eastern-European Journal of Enterprise Technologies, 3 (10 (87)), 63–73. doi: http://doi.org/10.15587/17294061.2017.102314
Pospelov, B., Rybka, E., Meleshchenko, R., Krainiukov, O., Harbuz, S., Bezuhla, Y. et. al. (2020). Use of uncertainty function for identification of hazardous states of atmospheric pollution vector. Eastern-European Journal of Enterprise Technologies, 2 (10 (104)), 6–12. doi: http://doi.org/10.15587/17294061.2020.200140
Sadkovyi, V., Pospelov, B., Andronov, V., Rybka, E., Krainiukov, O., Rud, A. et. al. (2020). Construction of a method for detecting arbitrary hazard pollutants in the atmospheric air based on the structural function of the current pollutant concentrations. Eastern-European Journal of Enterprise Technologies, 6 (10 (108)), 14–22. doi: http://doi.org/10.15587/17294061.2020.218714
Dubinin, D., Korytchenko, K., Lisnyak, A., Hrytsyna, I., Trigub, V. (2017). Numerical simulation of the creation of a fire fighting barrier using an explosion of a combustible charge. Eastern-European Journal of Enterprise Technologies, 6 (10 (90)), 11–16. doi: http://doi.org/10.15587/17294061.2017.114504
Danchenko, Y., Andronov, V., Kariev, A., Lebedev, V., Rybka, E., Meleshchenko, R., Yavorska, D. (2017). Research into surface properties of disperse fillers based on plant raw materials. Eastern-European Journal of Enterprise Technologies, 5 (12 (89)), 20–26. doi: http://doi.org/10.15587/17294061.2017.111350
Danchenko, Y., Andronov, V., Barabash, E., Obigenko, T., Rybka, E., Meleshchenko, R., Romin, A. (2017). Research of the intramolecular interactions and structure in epoxyamine composites with dispersed oxides. Eastern-European Journal of Enterprise Technologies, 6 (12 (90)), 4–12. doi: http://doi.org/10.15587/17294061.2017.118565
Otrosh, Y., Semkiv, O., Rybka, E., Kovalov, A. (2019). About need of calculations for the steel framework building in temperature influences conditions. IOP Conference Series: Materials Science and Engineering, 708 (1), 012065. doi: http://doi.org/10.1088/1757899x/708/1/012065
Dubinin, D., Korytchenko, K., Lisnyak, A., Hrytsyna, I., Trigub, V. (2018). Improving the installation for fire extinguishing with finelydispersed water. Eastern-European Journal of Enterprise Technologies, 2 (10 (92)), 38–43. doi: http://doi.org/10.15587/17294061.2018.127865
Kondratenko, O., Vambol, S., Strokov, O., Avramenko, A. (2015). Mathematical model of the efficiency of diesel particulate matter filter. Naukovyi Visnyk Natsionalnoho Hirnychoho Universytetu, 6, 55–61.
Semko, A., Beskrovnaya, M., Vinogradov, S., Hritsina, I., Yagudina, N. (2014). The usage of high speed impulse liquid jets for putting out gas blowouts. Journal of Theoretical and Applied Mechanics, 52 (3), 655–664.
Pospelov, B., Andronov, V., Rybka, E., Meleshchenko, R., Gornostal, S. (2018). Analysis of correlation dimensionality of the state of a gas medium at early ignition of materials. Eastern-European Journal of Enterprise Technologies, 5 (10 (95)), 25–30. doi: http://doi.org/10.15587/17294061.2018.142995
Panchenko, A., Voloshina, A., Boltyansky, O., Milaeva, I., Grechka, I., Khovanskyy, S. et. al. (2018). Designing the flowthrough parts of distribution systems for the PRG series planetary hydraulic motors. Eastern-European Journal of Enterprise Technologies, 3 (1 (93)), 67–77. doi: http://doi.org/10.15587/17294061.2018.132504
Pospelov, B., Andronov, V., Rybka, E., Popov, V., Romin, A. (2018). Experimental study of the fluctuations of gas medium parameters as early signs of fire. Eastern-European Journal of Enterprise Technologies, 1 (10 (91)), 50–55. doi: http://doi.org/10.15587/17294061.2018.122419
Vambol, S., Vambol, V., Bogdanov, I., Suchikova, Y., Rashkevich, N. (2017). Research of the influence of decomposition of wastes of polymers with nano inclusions on the atmosphere. Eastern-European Journal of Enterprise Technologies, 6 (10 (90)), 57–64. doi: http://doi.org/10.15587/17294061.2017.118213
Danchenko, Y., Andronov, V., Teslenko, M., Permiakov, V., Rybka, E., Meleshchenko, R., Kosse, A. (2018). Study of the free surface energy of epoxy composites using an automated measurement system. Eastern-European Journal of Enterprise Technologies, 1 (12 (91)), 9–17. doi: http://doi.org/10.15587/17294061.2018.120998
Otrosh, Y., Rybka, Y., Danilin, O., Zhuravskyi, M. (2019). Assessment of the technical state and the possibility of its control for the further safe operation of building structures of mining facilities. E3S Web of Conferences, 123, 01012. doi: http://doi.org/10.1051/e3sconf/201912301012
Andronov, V., Pospelov, B., Rybka, E., Skliarov, S. (2017). Examining the learning fire detectors under real conditions of application. Eastern-European Journal of Enterprise Technologies, 3 (9 (87)), 53–59. doi: http://doi.org/10.15587/17294061.2017.101985
Pospelov, B., Andronov, V., Rybka, E., Popov, V., Semkiv, O. (2018). Development of the method of frequencytemporal representation of fluctuations of gaseous medium parameters at fire. Eastern-European Journal of Enterprise Technologies, 2 (10 (92)), 44–49. doi: http://doi.org/10.15587/17294061.2018.125926
Pospelov, B., Andronov, V., Rybka, E., Meleshchenko, R., Borodych, P. (2018). Studying the recurrent diagrams of carbon monoxide concentration at early ignitions in premises. Eastern-European Journal of Enterprise Technologies, 3 (9 (93)), 34–40. doi: http://doi.org/10.15587/17294061.2018.133127
Andronov, V., Pospelov, B., Rybka, E. (2017). Development of a method to improve the performance speed of maximal fire detectors. Eastern-European Journal of Enterprise Technologies, 2 (9 (86)), 32–37. doi: http://doi.org/10.15587/17294061.2017.96694
Pospelov, B., Andronov, V., Rybka, E., Skliarov, S. (2017). Research into dynamics of setting the threshold and a probability of ignition detection by selfadjusting fire detectors. Eastern-European Journal of Enterprise Technologies, 5 (9 (89)), 43–48. doi: http://doi.org/10.15587/17294061.2017.110092
Osipchik, V. S., Yakovleva, R. A., Spirina, E. Y., Obizhenko, T. N., Rybko, E. A., Kondratenko, A. V. (2011). Influence of the Composition of the Redox System on the ThermoOxidative Degradation of Intercalated Graphites. International Polymer Science and Technology, 38 (1), 53–56. doi: http://doi.org/10.1177/0307174x1103800111
Pospelov, B., Andronov, V., Rybka, E., Skliarov, S. (2017). Design of fire detectors capable of selfadjusting by ignition. Eastern-European Journal of Enterprise Technologies, 4 (9 (88)), 53–59. doi: http://doi.org/10.15587/17294061.2017.108448
Abramov, Yu. A., Rybka, E. A., Gvozd, V. M. (2013). Termicheskii kompleks dlya ispytanii obraztsov stroitelnykh konstruktsii. Kharkiv: NUGZU, 128.
Pro zatverdzhennia Tekhnichnoho rehlamentu budivelnykh vyrobiv, budivel i sporud (2006). Postanova KMU No. 1764. 20.12.2006. Available at: https://zakon.rada.gov.ua/laws/show/17642006%D0%BF#Text
Ragimov, S., Sobyna, V., Vambol, S., Vambol, V., Feshchenko, A., Zakora, A. et. al. (2018). Physical modelling of changes in the energy impact on a worker taking into account hightemperature radiation. Journal of Achievements in Materials and Manufacturing Engineering, 1 (91), 27–33. doi: http://doi.org/10.5604/01.3001.0012.9654
Kustov, M., Slepuzhnikov, E., Lipovoy, V., Khmyrov, I., Dadashov, I. F., Buskin, O. (2019). Procedure for Implementation of the Method of Artificial Deposition of Radioactive Substances from the Atmosphere. Nuclear and Radiation Safety, 3 (83), 13–25. doi: http://doi.org/10.32918/nrs.2019.3(83).02
Dubinin, D., Cherkashyn, O., Maksymov, A., Beliuchenko, D., Hovalenkov, S., Shevchenko, S., Avetisyan, V. (2020). Investigation of the effect of carbon monoxide on people in case of fire in a building. Sigurnost, 62 (4), 347–357. doi: http://doi.org/10.31306/s.62.4.2
Otrosh, Y., Kovalov, A., Semkiv, O., Rudeshko, I., Diven, V. (2018). Methodology remaining lifetime determination of the building structures. MATEC Web of Conferences, 230, 02023. doi: http://doi.org/10.1051/matecconf/201823002023
Otrosh, Y., Surianinov, M., Golodnov, A., Starova, O. (2019). Experimental and Computer Researches of Ferroconcrete Beams at HighTemperature Influences. Materials Science Forum, 968, 355–360. doi: http://doi.org/10.4028/www.scientific.net/msf.968.355
Vasiliev, M. I., Movchan, I. O., Koval, O. M. (2014). Diminishing of ecological risk via optimization of fireextinguishing system projects in timberyards. Naukovyi Visnyk Natsionalnoho Hirnychoho Universytetu, 5, 106–113.
Popov, O., Iatsyshyn, A., Kovach, V., Artemchuk, V., Kameneva, I., Taraduda, D. et. al. (2020). Risk Assessment for the Population of Kyiv, Ukraine as a Result of Atmospheric Air Pollution. Journal of Health and Pollution, 10 (25). doi: http://doi.org/10.5696/2156961410.25.200303
Popov, O., Iatsyshyn, A., Kovach, V., Artemchuk, V., Taraduda, D., Sobyna, V. et. al. (2019). Physical Features of Pollutants Spread in the Air During the Emergency at NPPs. Nuclear and Radiation Safety, 4 (84), 88–98. doi: http://doi.org/10.32918/nrs.2019.4(84).11
Novak, S. V., Nefedchenko, L. M., Abramov, O. O. (2010). Metody vyprobuvan budivelnykh konstruktsii ta vyrobiv na vohnestiikist. Kyiv: Pozhinformtekhnika, 132.
Bashynska, O., Otrosh, Y., Holodnov, O., Tomashevskyi, A., Venzhego, G. (2020). Methodology for Calculating the Technical State of a ReinforcedConcrete Fragment in a Building Influenced by High Temperature. Materials Science Forum, 1006, 166–172. doi: http://doi.org/10.4028/www.scientific.net/msf.1006.166
Kovalov, A., Otrosh, Y., Rybka, E., Kovalevska, T., Togobytska, V., Rolin, I. (2020). Treatment of Determination Method for Strength Characteristics of Reinforcing Steel by Using Thread Cutting Method after Temperature Influence. Materials Science Forum, 1006, 179–184. doi: http://doi.org/10.4028/www.scientific.net/msf.1006.179
Pozdieiev, S., Nuianzin, O., Sidnei, S., Shchipets, S. (2017). Computational study of bearing walls fire resistance tests efficiency using different combustion furnaces configurations. MATEC Web of Conferences, 116, 02027. doi: http://doi.org/10.1051/matecconf/201711602027
Nuianzin, O., Pozdieiev, S., Hora, V., Shvydenko, A., Samchenko, T. (2018). Experimental study of temperature mode of a fire in a cable tunnel. Eastern-European Journal of Enterprise Technologies, 3 (10 (93)), 21–27. doi: http://doi.org/10.15587/17294061.2018.131792
Otrosh, Yu. A., Poklonskyi, V. H., Fesenko, O. A., Tarasiuk, V. H., Nemchynov, Yu. I., Baitala, Kh. Z. et. al. (2016). Rozrakhunok zalizobetonnykh konstruktsii na vohnestiikist vidpovidno do Yevrokodu 2. Kyiv: Intertekhnolohiia, 83.
Gravit, M., Golub, E. (2018). The fire resistant ceiling construction in a hydrocarbon fire. MATEC Web of Conferences, 245, 03004. doi: http://doi.org/10.1051/matecconf/201824503004
Vasilchenko, A., Doronin, E., Chernenko, O., Ponomarenko, I. (2019). Estimation of fire resistance of bending reinforced concrete elements based on concrete with disperse fibers. IOP Conference Series: Materials Science and Engineering, 708 (1), 012075. doi: http://doi.org/10.1088/1757899x/708/1/012075
Surianinov, M., Andronov, V., Otrosh, Y., Makovkina, T., Vasiukov, S. (2020). Concrete and Fiber Concrete Impact Strength. Materials Science Forum, 1006, 101–106. doi: http://doi.org/10.4028/www.scientific.net/msf.1006.101
Nekora, O., Slovynsky, V., Pozdieiev, S. (2017). The research of bearing capacity of reinforced concrete beam with use combined experimentalcomputational method. MATEC Web of Conferences, 116, 02024. doi: http://doi.org/10.1051/matecconf/201711602024
Pozdieiev, S., Nekora, O., Kryshtal, T., Zazhoma, V., Sidnei, S. (2018). Method of the calculated estimation of the possibility of progressive destruction of buildings in result of fire. MATEC Web of Conferences, 230, 02026. doi: http://doi.org/10.1051/matecconf/201823002026
Jiangtao, Y., Yichao, W., Kexu, H., Kequan, Y., Jianzhuang, X. (2017). The performance of nearsurface mounted CFRP strengthened RC beam in fire. Fire Safety Journal, 90, 86–94. doi: http://doi.org/10.1016/j.firesaf.2017.04.031
Roitman, V. M., Gabdulin, R. Sh. (2013). Obespechenie stoikosti zhelezobetonnykh konstruktsii protiv vzryvoobraznogo razrusheniya pri pozhare s pomoschyu tonkosloinykh ognezaschitnykh vspuchivayuschikhsya pokrytii. Pozhary i chrezvychainye situatsii: predotvraschenie, likvidatsiya, 213, 11–16.
Takla, M., Tarsha, I. (2020). Effect of temperature on carrying capacity of concrete columns confined with multilayers of CFRP. Jordan Journal of Civil Engineering, 14 (1), 14–26.
Vasilchenko, A., Doronin, E., Ivanov, B., Konoval, V. (2019). Effect of Residual Deformation of a Steel Column on its Fire Resistance under Combined Exposure “ExplosionFire.” Materials Science Forum, 968, 288–293. doi: http://doi.org/10.4028/www.scientific.net/msf.968.288
Korytchehko, K. V., Ozerov, A. N., Vinnikov, D. V., Skob, Y. A., Dubinin, D. P., Meleshchenko, R. G. (2018). Numerical simulation of influence of the nonequilibrium excitation of molecules on direct detonation initiation by spark discharge. Problems of Atomic Science and Technology, 116 (4), 194–199.
Skob, Y., Ugryumov, M., Dreval, Y. (2020). Numerical Modelling of Gas Explosion Overpressure Mitigation Effects. Materials Science Forum, 1006, 117–122. doi: http://doi.org/10.4028/www.scientific.net/msf.1006.117
Xue, C. (2020). Research on steel structure technology in civil engineering construction. IOP Conference Series: Earth and Environmental Science, 474 (7), 072072. doi: http://doi.org/10.1088/17551315/474/7/072072
Zhong, B., Zhang, Z., Ge, X., He, S., Huang, H. (2020). Development and Application of Fireproof Coatings for Fabricated Steel Structures. Material Sciences, 10 (12), 1027–1033. doi: http://doi.org/10.12677/ms.2020.1012121
Zhu, Y. Y., Zhao, D. L., He, Q., Zhang, J. X., Li, L. Y., Ma, D. D. (2014). Preparation and property of MCMB fireproof coating for steel structure. Gongneng Cailiao/Journal of Functional Materials, 45 (3), 03145–03148. doi: http://doi.org/10.3969/j.issn.10019731.2014.03.032
Xu, Q., Li, G.Q., Wang, Y. C., Bisby, L. (2020). An experimental study of the behavior of intumescent coatings under localized fires. Fire Safety Journal, 115, 103003. doi: http://doi.org/10.1016/j.firesaf.2020.103003
De Silva, D., Bilotta, A., Nigro, E. (2020). Approach for modelling thermal properties of intumescent coating applied on steel members. Fire Safety Journal, 116. doi: http://doi.org/10.1016/j.firesaf.2020.103200
Morys, M., Häßler, D., Krüger, S., Schartel, B., Hothan, S. (2020). Beyond the standard timetemperature curve: Assessment of intumescent coatings under standard and deviant temperature curves. Fire Safety Journal, 112, 102951. doi: http://doi.org/10.1016/j.firesaf.2020.102951
Bychkov, A. S., Kondratiev, A. V. (2019). CriterionBased Assessment of Performance Improvement for Aircraft Structural Parts with Thermal Spray Coatings. Journal of Superhard Materials, 41 (1), 53–59. doi: http://doi.org/10.3103/s1063457619010088
Wang, L., Chen, B., Zhang, C., Li, G. (2019). Experimental study on insulative properties of intumescent coating exposed to standard and nonstandard furnace curves. Fire and Materials, 43 (7), 782–793. doi: http://doi.org/10.1002/fam.2737
Maciulaitis, R., Grigonis, M., Malaiskiene, J. (2018). The impact of the aging of intumescent fire protective coatings on fire resistance. Fire Safety Journal, 98, 15–23. doi: http://doi.org/10.1016/j.firesaf.2018.03.007
Tian, N., Delichatsios, M. A., Zhang, J., Fateh, T. (2018). A methodology and a simple engineering fire performance model for Intumescent Fire Retardant coatings. Fire Safety Journal, 98, 120–129. doi: http://doi.org/10.1016/j.firesaf.2018.04.010
Pozdieiev, S., Nuianzin, O., Borsuk, O., Nedilko, I. (2021). Research of Integrity of Fire Insulation Cladding with Mineral Wool of Steel Beam under Fire Impact. IOP Conference Series: Materials Science and Engineering, 1021 (1), 012024. doi: http://doi.org/10.1088/1757899x/1021/1/012024
Dreyer, J. A. H., Weinell, C. E., DamJohansen, K., Kiil, S. (2021). Review of heat exposure equipment and insitu characterisation techniques for intumescent coatings. Fire Safety Journal, 121, 103264. doi: http://doi.org/10.1016/j.firesaf.2020.103264
Lucherini, A., Giuliani, L., Jomaas, G. (2018). Experimental study of the performance of intumescent coatings exposed to standard and nonstandard fire conditions. Fire Safety Journal, 95, 42–50. doi: http://doi.org/10.1016/j.firesaf.2017.10.004
Roitman, V. M. (2006). Inzhenernye aspekty sobytii 11 sentyabrya 2001 goda v NyuIorke pri atake terroristami bashen Vsemirnogo torgovogo tsentra. Globalnaya bezopasnost, 3, 30–35.
De Souza, R. C. S., Andreini, M., La Mendola, S., Zehfuß, J., Knaust, C. (2019). Probabilistic thermomechanical finite element analysis for the fire resistance of reinforced concrete structures. Fire Safety Journal, 104, 22–33. doi: http://doi.org/10.1016/j.firesaf.2018.12.005
Rastorguev, B. S., Plotnikov, A., Khusnutdinov, D. Z. (2007). Proektirovanie zdanii i sooruzhenii pri avariinykh vzryvnykh vozdeistviyakh. Izdatelstvo Assotsiatsii stroitelnykh vuzov, 154.
Pershakov, V. M., Barabash, M. S., Bieliatynskyi, A. O., Lysnytska, K. M. (2015). Problemy protydii konstruktsii prohresuiuchomu obvalenniu budivel ta sporud. Kyiv, 456.
Manco Rivera, M. R., Vaz, M. A., Cyrino, J. C. R., Landesmann, A. (2021). A study on the use of fireproof protection in offshore topside steel structures subject to localised fires. Marine Systems & Ocean Technology, 16 (2), 55–68. doi: http://doi.org/10.1007/s4086802100102x
Bolina, F. L., Prager, G. L., Rodrigues, E., Tutikian, B. F. (2015). Evaluation of fire resistance of massive reinforced concrete walls. Ambiente Construído, 15 (4), 291–305. doi: http://doi.org/10.1590/s167886212015000400051
Lucherini, A., Maluk, C. (2019). Assessing the onset of swelling for thin intumescent coatings under a range of heating conditions. Fire Safety Journal, 106, 1–12. doi: http://doi.org/10.1016/j.firesaf.2019.03.014
Zeng, Y., Weinell, C. E., DamJohansen, K., Ring, L., Kiil, S. (2020). Comparison of an industrial and a laboratoryscale furnace for analysis of hydrocarbon intumescent coating performance. Journal of Fire Sciences, 38 (3), 309–329. doi: http://doi.org/10.1177/0734904120902852
Mariappan, T. (2016). Recent developments of intumescent fire protection coatings for structural steel: A review. Journal of Fire Sciences, 34 (2), 120–163. doi: http://doi.org/10.1177/0734904115626720
Zavyalov, D. E. (2013). Povyshenie effektivnosti ognezaschitnykh vspuchivayuschikhsya kompozitsii. Saint Petersburg, 118.
Cirpici, B. K., Wang, Y. C., Rogers, B. (2016). Assessment of the thermal conductivity of intumescent coatings in fire. Fire Safety Journal, 81, 74–84. doi: http://doi.org/10.1016/j.firesaf.2016.01.011
Gillet, M., Perez, L., Autrique, L. (2019). A model based predictive tool for fire safety intumescent coatings design. Fire Safety Journal, 110, 102908. doi: http://doi.org/10.1016/j.firesaf.2019.102908
Novak, S. V., Drizhd, V. L., Dobrostan, O. V. (2018). Analysis of modern european methods of evaluation of fireproof ability of fireproof materials for building constructions. Naukovyi visnyk: tsyvilnyi zakhyst ta pozhezhna bezpeka, 5 (1), 74–85.
Michel, M. A., Tutikian, B. F., Ortolan, V., Oliveira, M. L. S., Sampaio, C. H., Gómez P, L., Silva, L. F. O. (2019). Fire resistance performance of concretePVC panels with polyvinyl chloride (PVC) stay in place (SIP) formwork. Journal of Materials Research and Technology, 8 (5), 4094–4107. doi: http://doi.org/10.1016/j.jmrt.2019.07.018
Michel Murillo, A., Valery Abisambra, G., Aura Acosta, P., Claudia Quesada, Q., Tutikian, B. F., Ehrenbring, H. Z. (2021). Comparison of the fire resistance behaviour of structural insulated panels with expanded polystyrene core treated with intumescent coating. Journal of Materials Research and Technology, 12, 1958–1969. doi: http://doi.org/10.1016/j.jmrt.2021.03.079
Zhang, T., Zhang, Y., Xiao, Z., Yang, Z., Zhu, H., Ju, J. W., Yan, Z. (2019). Development of a novel bioinspired cementbased composite material to improve the fire resistance of engineering structures. Construction and Building Materials, 225, 99–111. doi: http://doi.org/10.1016/j.conbuildmat.2019.07.121
Beh, J. H., Yew, M. C., Saw, L. H. (2020). Development of lightweight fire resistant sandwich panel. IOP Conference Series: Earth and Environmental Science, 476 (1), 012031. doi: http://doi.org/10.1088/17551315/476/1/012031
Shepel, S. V., Wakili, K. G., Hugi, E. (2012). Investigation of heat transfer in gypsum plasterboard exposed to fire for three nominal fire scenarios. Journal of Fire Sciences, 30 (3), 240–255. doi: http://doi.org/10.1177/0734904111433265
Medri, V., Papa, E., Mazzocchi, M., Laghi, L., Morganti, M., Francisconi, J., Landi, E. (2015). Production and characterization of lightweight vermiculite/geopolymerbased panels. Materials & Design, 85, 266–274. doi: http://doi.org/10.1016/j.matdes.2015.06.145
Gulіda, E. M., Renkas, A. A. (2012). The fireresistant of concreteslabs in residential and administrative buildings. Problemy pozharnoi bezopasnosti, 32, 62–73.
Strakhov, V. L., Krutov, A. M., Davydkin, N. F. (2000). Ognezaschita stroitelnykh konstruktsii. Moscow: TIMR, 433.
Chernukha, A., Teslenko, A., Kovalov, P., Bezuglov, O. (2020). Mathematical Modeling of FireProof Efficiency of Coatings Based on Silicate Composition. Materials Science Forum, 1006, 70–75. doi: http://doi.org/10.4028/www.scientific.net/msf.1006.70
Skorodumova, O., Tarakhno, O., Chebotaryova, O., Hapon, Y., Emen, F. M. (2020). Formation of Fire Retardant Properties in Elastic Silica Coatings for Textile Materials. Materials Science Forum, 1006, 25–31. doi: http://doi.org/10.4028/www.scientific.net/msf.1006.25
Kireev, A., Tregubov, D., Safronov, S., Saveliev, D. (2020). Study Insulating and Cooling Properties of the Material on the Basis of Crushed Foam Glass and Determination of its Extinguishing Characteristics with the Attitude to Alcohols. Materials Science Forum, 1006, 62–69. doi: http://doi.org/10.4028/www.scientific.net/msf.1006.62
Puri, R. G., Khanna, A. S. (2016). Intumescent coatings: A review on recent progress. Journal of Coatings Technology and Research, 14 (1), 1–20. doi: http://doi.org/10.1007/s1199801698153
Xu, Q., Li, G.Q., Jiang, J., Wang, Y. C. (2018). Experimental study of the influence of topcoat on insulation performance of intumescent coatings for steel structures. Fire Safety Journal, 101, 25–38. doi: http://doi.org/10.1016/j.firesaf.2018.08.006
Li, G.Q., Han, J., Lou, G.B., Wang, Y. C. (2016). Predicting intumescent coating protected steel temperature in fire using constant thermal conductivity. ThinWalled Structures, 98, 177–184. doi: http://doi.org/10.1016/j.tws.2015.03.008
Tsoi, A. A., Demekhin, F. V. (2016). Criteria of model of fire rating of flameretardant coating for steel structures in the conditions of hydrocarbon jet fire. Mezhdunarodnyi nauchnoissledovatelskii zhurnal, 6–2 (48), 161–165.
Holodnov, O. I., Antoshyna, T. V., Otrosh, Yu. A. (2017). About necessity of calculation of buildingswith steel framework on thermalactions. Zbirnyk naukovykh prats Ukrainskoho instytutu stalevykh konstruktsii imeni V. M. Shymanovskoho, 20, 65–84.
Gravit, M., Gumerova, E., Bardin, A., Lukinov, V. (2017). Increase of fire resistance limits of building structures of oilandgas complex under hydrocarbon fire. Energy Management of Municipal Transportation Facilities and Transport. Cham: Springer, 818–829. doi: http://doi.org/10.1007/9783319709871_87
Imran, M., Liew, M. S., Nasif, M. S., Niazi, U. M., Yasreen, A. (2017). Hazard Assessment Studies on Hydrocarbon Fire and Blast: An Overview. Advanced Science Letters, 23 (2), 1243–1247. doi: http://doi.org/10.1166/asl.2017.8349
Abramov, I. V., Gravit, M. V., Gumerova, E. I. (2018). Increase in the fire resistance limits of ship and building structures with hydrocarbon fire. Gazovaya promyshlennost, 5 (768), 106–115.
Lucherini, A., Maluk, C. (2019). Intumescent coatings used for the firesafe design of steel structures: A review. Journal of Constructional Steel Research, 162, 105712. doi: http://doi.org/10.1016/j.jcsr.2019.105712
Sobur, S. V. (2008). Ognezaschita materialov i konstruktsii. Moscow: Izdatelstvo “PozhKniga”, 216.
Wang, L., Wang, Y., Zeng, W. (2021). An experimental study on crack and debonding of intumescent coatings and their effects on temperature development of steel elements. Fire Safety Journal, 122, 103325. doi: http://doi.org/10.1016/j.firesaf.2021.103325
Yew, M. C., Ramli Sulong, N. H. (2012). Fireresistive performance of intumescent flameretardant coatings for steel. Materials & Design, 34, 719–724. doi: http://doi.org/10.1016/j.matdes.2011.05.032
Wang, L. L., Wang, Y. C., Li, G. Q., Zhang, Q. Q. (2020). An experimental study of the effects of topcoat on aging and fire protection properties of intumescent coatings for steel elements. Fire Safety Journal, 111, 102931. doi: http://doi.org/10.1016/j.firesaf.2019.102931
Fateh, T., Guillaume, E., Joseph, P. (2017). An experimental study of the thermal performance of a novel intumescent fire protection coating. Fire Safety Journal, 92, 132–141. doi: http://doi.org/10.1016/j.firesaf.2017.05.021
Yamschikova, S. A. (2009). Povyshenie ognezaschitnoi sposobnosti vspuchivayuschikhsya pokrytii dlya obektov neftegazovoi otrasli. Ufa, 171.
Vakhitova, L., Taran, N., Bessarabov, V., Drizhd, V., Pridatko, S., Prudchenko, A. (2018). Development of fire retardant coating for the conditions of hydrocarbon fire with improved rheological characteristics. Journal of Donetsk Mining Institute, 1 (42), 103–115. doi: http://doi.org/10.31474/1999981x20181103115
Beh, J. H., Yew, M. C., Yew, M. K., Saw, L. H. (2019). Fire Protection Performance and Thermal Behavior of Thin Film Intumescent Coating. Coatings, 9 (8), 483. doi: http://doi.org/10.3390/coatings9080483
Wang, Y., Zhao, J. (2019). Facile preparation of slag or fly ash geopolymer composite coatings with flame resistance. Construction and Building Materials, 203, 655–661. doi: http://doi.org/10.1016/j.conbuildmat.2019.01.097
M. Beraldo, C. H., da S. Silveira, M. R., F. Baldissera, A., Ferreira, C. A. (2019). A new benzoxazinebased intumescent coating for passive protection against fire. Progress in Organic Coatings, 137, 105321. doi: http://doi.org/10.1016/j.porgcoat.2019.105321
Inerhunwa, I., Wang, Y. C., Su, M. (2019). Reliability analysis of intumescent coating protected steel members under the standard fire condition. Fire Safety Journal, 104, 43–56. doi: http://doi.org/10.1016/j.firesaf.2018.12.003
Pignatta e Silva, V. (2005). Determination of the steel fire protection material thickness by an analytical process – a simple derivation. Engineering Structures, 27 (14), 2036–2043. doi: http://doi.org/10.1016/j.engstruct.2005.05.018
ENV 133814: 2002 Test methods for determining the contribution to the fire resistance of structural members – Part 4: Applied protection to steel members (2002). Available at: https://standards.iteh.ai/catalog/standards/cen/5463307067554b788818bb6d3fa92eb7/env1338142002
Alcaíno, P., SantaMaría, H., MagnaVerdugo, C., López, L. (2020). Experimental fastassessment of postfire residual strength of reinforced concrete frame buildings based on nondestructive tests. Construction and Building Materials, 234, 117371. doi: http://doi.org/10.1016/j.conbuildmat.2019.117371
Nair, A., Salem, O. (Sam). (2020). Experimental determination of the residual compressive strength of concrete columns subjected to different fire durations and load ratios. Journal of Structural Fire Engineering, 11 (4), 529–543. doi: http://doi.org/10.1108/jsfe1020190034
Kuehnen, R., Youssef, M., ElFitiany, S. (2020). Performancebased design of RC beams using an equivalent standard fire. Journal of Structural Fire Engineering, 12 (1), 98–109. doi: http://doi.org/10.1108/jsfe0220200008
Bai, L. L., Song, T. (2012). Failure Analysis of Reinforced Concrete Columns after High Temperature. Applied Mechanics and Materials, 157158, 1578–1581. doi: http://doi.org/10.4028/www.scientific.net/amm.157158.1578
Novak, S., Drizhd, V., Dobrostan, O., Maladyka, L. (2019). Influence of testing samples’ parameters on the results of evaluating the fireprotective capability of materials. Eastern-European Journal of Enterprise Technologies, 2 (10 (98)), 35–42. doi: http://doi.org/10.15587/17294061.2019.164743
De Silva, D., Bilotta, A., Nigro, E. (2019). Experimental investigation on steel elements protected with intumescent coating. Construction and Building Materials, 205, 232–244. doi: http://doi.org/10.1016/j.conbuildmat.2019.01.223
Nadjai, A., Petrou, K., Han, S., Ali, F. (2016). Performance of unprotected and protected cellular beams in fire conditions. Construction and Building Materials, 105, 579–588. doi: http://doi.org/10.1016/j.conbuildmat.2015.12.150
Novak, S. V. (2016). Parameters reasoning of samples for experimental determination of the temperature of the steel plates that are fireretardant coating in conditions of fire exposure under standard temperature fire regime. Naukovyi visnyk: Tsyvilnyi zakhyst ta pozhezhna bezpeka, 2, 18–24.
Novak, S. V., Krukovskyi, P. H., Perepylytsia, M. S. (2016). Determination of the temperature distribution in steel construction in terms of fire exposure calculation methods. Naukovyi visnyk: tsyvilnyi zakhyst ta pozhezhna bezpeka, 1, 9–15.
Lakhani, H., Kamath, P., Bhargava, P., Sharma, U., Reddy, G. (2013). Thermal Analysis of Reinforced Concrete Structural Elements. Journal of Structural Fire Engineering, 4 (4), 227–244. doi: http://doi.org/10.1260/20402317.4.4.227
Garlińska, U., Michalak, P., Popielarczyk, T. (2015). Szacowanie możliwości utraty nośności konstrukcji budowlanej w warunkach pożaru. Bezpieczeństwo i Technika Pożarnicza, 39 (3), 59–66. doi: http://doi.org/10.12845/bitp.39.3.2015.5
Biskupska, N., Szymkuć, W. (2016). Towards the simplified modelling of thermal and mechanical response of steel tubular columns exposed to localized fire. Modern Building Materials, Structures and Techniques.
Zárate, L. G., Lara, H. E., Cordero, M. E., Kozanoglu, B. (2014). Infrared thermography and CFD analysis of hydrocarbon jet fires. Chemical Engineering Transactions, 39, 1357–1362. doi: http://doi.org/10.3303/CET1439227
Golodnov, A. I., Kudryashov, V. A., Polevoda, I. I., Otrosh, Yu. A., Tkachuk, I. A., Seminog, N. N., Drobysh, A. S. (2015). Sopostavitelnaya otsenka ognestoikosti zhelezobetonnykh mnogopustotnykh plit s ispolzovaniem standartov Belarusi, Ukrainy, Evropeiskogo Soyuza, a takzhe raschetnykh metodov. Vestnik Universiteta grazhdanskoi zaschity MCHS Belarusi, 1 (21), 30–39.
Džolev, I., Cvetkovska, M., Radonjanin, V., Lađinović, Đ., Laban, M. (2018). Modelling approach of structural fire performance. Book of proceedings, 17–24.
Pozdieiev, S. V., Otrosh, Yu. A., Demeshok, V. V., Fedchenko, I. V. (2016). Rozrakhunkovyi metod otsinky vohnestiikosti plyty perekryttia. Promyslove budivnytstvo ta inzhenerni sporudy, 2, 28–33.
Pozdieiev, S. V., Otrosh, Yu. A., Omelchenko, A. M., Kropyva, M. O. (2015). Temperature fields interpretation method in the cross section of reinforced concrete beams. Zbirnyk naukovykh prats Poltavskoho natsionalnoho tekhnichnoho universytetu im. Yu. Kondratiuka. Seriia: Haluzeve mashynobuduvannia, budivnytstvo, 2, 70–78.
Pozdeev, S. V. (2011). Verification of results of the specified calculation method of determination of limits of fireresistance of reinforced concrete constructions. Problemy pozharnoi bezopasnosti, 29, 141–148.
AlosMoya, J., PayaZaforteza, I., Garlock, M. E. M., LomaOssorio, E., Schiffner, D., Hospitaler, A. (2014). Analysis of a bridge failure due to fire using computational fluid dynamics and finite element models. Engineering Structures, 68, 96–110. doi: http://doi.org/10.1016/j.engstruct.2014.02.022
Džolev, I., Radujković, A., Cvetkovska, M., Lađinović, Đ., Radonjanin, V. (2016). Fire analysis of a simply supported steel beam using Opensees and Ansys Workbench. 4th International Conference Contemporary Achievements in Civil Engineering. Subotica, 315–322. doi: http://doi.org/10.14415/konferencijagfs2016.031
Łukomski, M., Turkowski, P., Roszkowski, P., Papis, B. (2017). Fire Resistance of Unprotected Steel Beams – Comparison between Fire Tests and Calculation Models. Procedia Engineering, 172, 665–672. doi: http://doi.org/10.1016/j.proeng.2017.02.078
Lin, S., Huang, Z., Fan, M. (2015). The effects of protected beams and their connections on the fire resistance of composite buildings. Fire Safety Journal, 78, 31–43. doi: http://doi.org/10.1016/j.firesaf.2015.08.003
Lazarevska, M., Cvetkovska, M., Knežević, M., Trombeva Gavriloska, A., Milanovic, M., Murgul, V., Vatin, N. (2014). Neural Network Prognostic Model for Predicting the Fire Resistance of Eccentrically Loaded RC Columns. Applied Mechanics and Materials, 627, 276–282. doi: http://doi.org/10.4028/www.scientific.net/amm.627.276
Zhang, X., Xiao, Y., Kunnath, S. K. (2015). State of the art and prospect of research on fire resistance behavior of reinforced concrete columns. Journal of Natural Disasters, 24 (3), 120–131. doi: http://doi.org/10.13577/j.jnd.2015.0316
EN 199212:2004 Eurocode 2: Design of concrete structures – Part 12: General rules – Structural fire design (2004). Available at: https://www.phd.eng.br/wpcontent/uploads/2015/12/en.1992.1.2.2004.pdf
EN 13632: 1999 Fire resistance tests – Part 2: Alternative and additional procedures (1999). Availablr at: https://standards.iteh.ai/catalog/standards/cen/2c26aa21abe74d9696a2eb8fbb4919e0/en136321999
Ng, Y. H., Zope, I. S., Dasari, A., Tan, K. H. (2020). Correlating the Performance of a FireRetardant Coating across Different Scales of Testing. Polymers, 12 (10), 2271. doi: http://doi.org/10.3390/polym12102271
Paik, J. K., Czujko, J. (2012). Engineering and design disciplines associated with management of hydrocarbon explosion and fire risks in offshore oil and gas Facilities. doi: http://doi.org/10.5957/smc2012a24
Paik, J. K., Czujko, J. (2011). Assessment of hydrocarbon explosion and fire risks in offshore installations: recent advances and future trends. The IES Journal Part A: Civil & Structural Engineering, 4 (3), 167–179. doi: http://doi.org/10.1080/19373260.2011.593345
Paik, J. K., Kim, B. J., Jeong, J. S., Kim, S. H., Jang, Y. S., Kim, G. S. et. al. (2010). CFD simulations of gas explosion and fire actions. Ships and Offshore Structures, 5 (1), 3–12. doi: http://doi.org/10.1080/17445300902872028
Uribe, S., Zárate, L., SuoAnttila, A., Cordero, M. E., Smith, J. D. (2020). Improvement in the prediction of gasoline pool fire behaviour: CFD modelling and validation. Journal of Loss Prevention in the Process Industries, 68, 104317. doi: http://doi.org/10.1016/j.jlp.2020.104317
PayáZaforteza, I., Garlock, M. E. M. (2010). A 3D numerical analysis of a typical steel highway overpass bridge under a hydrocarbon fire. Structures in Fire – Proceedings of the Sixth International Conference, SiF’10, 11–18.
ZVTTunnel – Zusatzliche techniche Vertragsbedingungen und Richtlinien fur den Bau von Strassentunneln. Tel. 1,2,4. Ausgabe, 1995 und 2000.
Subota, A. V., Semerak, M. M., Stokaliuk, O. V. (2014). Definition and study of the temperature field in the elements of metal structure in hydrogen combustion temperature mode. Pozhezhna bezpeka, 24, 120–123.
Yakymenko, O. P. (2012). Pidvyshchennia vohnestiikosti zalizobetonnykh oprav tunelnykh sporud. Kyiv, 141.
Enaleev, R. Sh., Telyakov, E. Sh., Tuchkova, O. A., Kachalkin, A. V., Osipova, L. E. (2010). Ognestoikost elementov stroitelnykh konstruktsii pri pozharakh v neftegazovom komplekse. Butlerovskie soobscheniya, 19 (3), 66–75.
Tuchkova, O. A. (2011). Gradientnotemperaturnyi kriterii ognestoikosti betonnykh konstruktsii pri pozharakh v khimicheskoi otrasli promyshlennosti. Kazan, 117.
Calabrese, L., Cattani, L., Vocale, P. (2014). Parameter estimation approach applied to the characterization of an intumescent fire retardant paint. JP Journal of Heat and Mass Transfer, 9 (2), 101–116.
Zehfuß, J., Sander, L., Schaumann, P., Weisheim, W. (2018). Thermische Materialeigenschaften von Brandschutzmaterialien für Naturbrandbeanspruchungen. Bautechnik, 95 (8), 535–546. doi: http://doi.org/10.1002/bate.201800033
Weisheim, W., Schaumann, P., Sander, L., Zehfuß, J. (2020). Numerical model for the fire protection performance and the design of intumescent coatings on structural steel exposed to natural fires. Journal of Structural Fire Engineering, 11 (1), 33–50. doi: http://doi.org/10.1108/jsfe0120190004
Yasir, M., Ahmad, F., Yusoff, P. S. M. M., Ullah, S., Jimenez, M. (2019). Latest trends for structural steel protection by using intumescent fire protective coatings: a review. Surface Engineering, 36 (4), 334–363. doi: http://doi.org/10.1080/02670844.2019.1636536
Wang, L., Dong, Y., Zhang, C., Zhang, D. (2015). Experimental Study of Heat Transfer in Intumescent Coatings Exposed to NonStandard Furnace Curves. Fire Technology, 51 (3), 627–643. doi: http://doi.org/10.1007/s1069401504607
STB 11.03.022010. Fire safety standards system. Fire protection. General technical requirements and test methods (2010). Available at: https://files.stroyinf.ru/Index2/1/4293725/4293725240.htm
GOST 2857490 Zaschita ot korrozii v stroitelstve. Konstruktsii betonnye i zhelezobetonnye. Metody ispytanii adgezii zaschitnykh pokrytii. Vved. 01.01.1991 (2004). Moscow: Standartinform, 7.
GOST 1514078 Materialy lakokrasochnye. Metody opredeleniya adgezii. Vved. 01.01.1979 (1990). Moscow: Gosudarstvennyi komitet SSSR po standartam, 12.
ETAG No. 0181:2004 Guide for the European technical approval of fire protective products – Part 1: General (2004). Available at: https://www.itb.pl/g/f/NDYz
ETAG No. 0182:2013 Guide for the European technical approval of fire protective products – Part 2: Reactive coatings for fire protection of steel elements (2013). Available at: https://www.nlfnorm.cz/en/ehn/6383
ETAG No. 0183:2013 Guide for the European technical approval of fire protective products – Part 3: Renderings and rendering kits intended for fire resisting applications. Available at: https://www.itb.pl/g/f/NDY1
ETAG No. 0184:2011 Guide for the European technical approval of fire protective products – Part 4: Fire protective board, slab and mat products and kits. Available at: https://www.eota.eu/sites/default/files/uploads/ETAGs/etag018part4ecversiondecember2011.pdf
GOST 9.401–91. Pokrytiya lakokrasochnye. Obschie trebovaniya i metody uskorennykh ispytanii na stoikost k vozdeistviyu klimaticheskikh faktorov. Data vvedeniya 19920701 (1991). Moscow: MKHINP, 55.
Jimenez, M., Bellayer, S., Revel, B., Duquesne, S., Bourbigot, S. (2013). Comprehensive Study of the Influence of Different Aging Scenarios on the Fire Protective Behavior of an Epoxy Based Intumescent Coating. Industrial & Engineering Chemistry Research, 52 (2), 729–743. doi: http://doi.org/10.1021/ie302137g
Zybina, O., Gravit, M., Stein, Y. (2017). Influence of carbon additives on operational properties of the intumescent coatings for the fire protection of building constructions. IOP Conference Series: Earth and Environmental Science, 90, 012227. doi: http://doi.org/10.1088/17551315/90/1/012227
Ustinov, A., Zybina, O., Tomakhova, A., Pavlov, S. (2018). The enhancement of operating properties of intumescent fireprotective compositions. MATEC Web of Conferences, 245, 11008. doi: http://doi.org/10.1051/matecconf/201824511008
Zubielewicz, M., Langer, E., Królikowska, A. (2021). Trends in the development of intumescent paints for the protection of steel structures and new related with them expectations. Ochrona Przed Korozja, 1 (7), 4–12. doi: http://doi.org/10.15199/40.2021.7.1
Wang, L. L., Wang, Y. C., Li, G. Q. (2013). Experimental study of hydrothermal aging effects on insulative properties of intumescent coating for steel elements. Fire Safety Journal, 55, 168–181. doi: http://doi.org/10.1016/j.firesaf.2012.10.004
Wang, L. L., Wang, Y. C., Yuan, J. F., Li, G. Q. (2012). Thermal conductivity of intumescent coating char after accelerated aging. Fire and Materials, 37 (6), 440–456. doi: http://doi.org/10.1002/fam.2137
Wang, J. (2016). The protective effects and aging process of the topcoat of intumescent fireretardant coatings applied to steel structures. Journal of Coatings Technology and Research, 13 (1), 143–157. doi: http://doi.org/10.1007/s1199801597339
Roberts, T. A., Shirvill, L. C., Waterton, K., Buckland, I. (2010). Fire resistance of passive fire protection coatings after longterm weathering. Process Safety and Environmental Protection, 88 (1), 1–19. doi: http://doi.org/10.1016/j.psep.2009.09.003
Dong, Y., Wang, G., Su, Q. (2014). Influence of degree of polymerization of ammonium polyphosphate on antiaging property of waterborne fire resistive coatings. Surface and Coatings Technology, 246, 71–76. doi: http://doi.org/10.1016/j.surfcoat.2014.03.009
Çırpıcı, B. K., Orhan, S. N., Kotan, T. (2019). Numerical modelling of heat transfer through protected composite structural members. Challenge Journal of Structural Mechanics, 5 (3), 96. doi: http://doi.org/10.20528/cjsmec.2019.03.003
Elliott, A., Temple, A., Maluk, C., Bisby, L. (2014). Novel Testing to Study the Performance of Intumescent Coatings under NonStandard Heating Regimes. Fire Safety Science, 11, 652–665. doi: http://doi.org/10.3801/iafss.fss.11652
Lucherini, A., Maluk, C. (2017). Novel test methods for studying the fire performance of thin intumescent coatings. Proceedings of 2nd International Fire Safety Symposium (IFireSS). Napoli, 565–572.
Li, G., Han, J., Wang, Y. C. (2017). Constant effective thermal conductivity of intumescent coatings: Analysis of experimental results. Journal of Fire Sciences, 35 (2), 132–155. doi: http://doi.org/10.1177/0734904117693857
Kolšek, J., Češarek, P. (2015). Performancebased fire modelling of intumescent painted steel structures and comparison to EC3. Journal of Constructional Steel Research, 104, 91–103. doi: http://doi.org/10.1016/j.jcsr.2014.10.008
Bozzoli, F., Mocerino, A., Rainieri, S., Vocale, P. (2018). Inverse heat transfer modeling applied to the estimation of the apparent thermal conductivity of an intumescent fire retardant paint. Experimental Thermal and Fluid Science, 90, 143–152. doi: http://doi.org/10.1016/j.expthermflusci.2017.09.006
Kraus, P., Mensinger, M., Tabeling, F., Schaumann, P. (2015). Experimental and Numerical Investigations of Steel Profiles with Intumescent Coating Adjacent to SpaceEnclosing Elements in Fire. Journal of Structural Fire Engineering, 6 (4), 237–246. doi: http://doi.org/10.1260/20402317.6.4.237
Standard for Safety for Rapid Rise Fire Tests of Protection Materials for Structural Steel: ANSI/UL 1709 (2007). Available at: https://ua1lib.org/book/14973256/24a856
Standard Methods of Fire Endurance Tests of Building Construction and Materials: CAN/ULCS10104. Available at: https://www.scc.ca/en/standardsdb/standards/19257
Gong, J., Xiang, Q., Zhao, M. (2014). Laboratory Research on Composite Tunnel FireRetardant Coating. Materials and Manufacturing Processes, 30 (6), 699–705. doi: http://doi.org/10.1080/10426914.2014.941872
Zhang, Y., Wang, Y. C., Bailey, C. G., Taylor, A. P. (2012). Global modelling of fire protection performance of an intumescent coating under different furnace fire conditions. Journal of Fire Sciences, 31 (1), 51–72. doi: http://doi.org/10.1177/0734904112453566
Andronov, V., Pospelov, B., Rybka, E. (2016). Increase of accuracy of definition of temperature by sensors of fire alarms in real conditions of fire on objects. Eastern-European Journal of Enterprise Technologies, 4 (5 (82)), 38–44. doi: http://doi.org/10.15587/17294061.2016.75063
Maliarenko, V. A., Redko, A. F., Chaika, Yu. Y., Povolochko, V. B. (2001). Tekhnichna teplofizyka ohorodzhuiuchykh konstruktsii budivel ta sporud. Kharkiv: Rubikon, 280.
Kovalov, A. I. (2017). Justification of parameters of fire protective plaster of coating for protecting concrete structures. Naukovyi visnyk: tsyvilnyi zakhyst ta pozhezhna bezpeka, 1 (3), 20–27.
Krukovskii, P. G., Kovalev, A. I., CHernenko, K. A., Metel, M. A., Abramov, A. A. (2012). Modelling of thermal state and fireresistance quality of hollow core armoured concrete floors. Pozharnaya bezopasnost, 21, 85–94.
Kovalov, A., Otrosh, Y., Ostroverkh, O., Hrushovinchuk, O., Savchenko, O. (2018). Fire resistance evaluation of reinforced concrete floors with fireretardant coating by calculation and experimental method. E3S Web of Conferences, 60, 00003. doi: http://doi.org/10.1051/e3sconf/20186000003
Kovalov, A., Konoval, V., Khmyrova, A., Dudko, K. (2019). Parameters for simulation of the thermal state and fireresistant quality of hollowcore floors used in the mining industry. E3S Web of Conferences, 123, 01022. doi: http://doi.org/10.1051/e3sconf/201912301022
Kovalev, A. I. (2013). Opredelenie kharakteristiki ognezaschitnoi sposobnosti pokrytii zhelezobetonnykh perekrytii dlya razlichnykh temperaturnykh rezhimov pozhara. Pozhezhna bezpeka: teorіya і praktika, 13, 4–9.
Kovalev, A. I. (2013). Vliyanie parametrov modeli na tochnost opredeleniya teplofizicheskikh kharakteristik ognezaschitnogo pokrytiya. Pozhezhna bezpeka: teorіya і praktika, 14, 64–68.
Kovalev, A. I., Krukovskii, P. G., Kachkar, E. V. (2012). Opredelenie kharakteristik vspuchivayuschegosya ognezaschitnogo pokrytiya «Feniks STV» po dannym ispytanii na ognestoikost monolitnogo perekrytiya. Pozhezhna bezpeka: teorіya і praktika, 11, 43–51.
Kovalev, A. Y. (2012). Evaluation of fire resistance rating of multihollow ferroconcrete floors with fireretardant coatings by experimentcalculation method. Naukovyi visnyk UkrNDIPB, 2, 28–34.
Kovalov, A. І., Otrosh, Yu. A., Danіlіn, O. M. (2019). Experimental studies of fire resistance of reinforced concrete floors with fire protection system. Problemy pozharnoi bezopasnosti, 45, 73–78.
Alifanov, O. M. (1988). Obratnye zadachi teploobmena. Moscow: Mashinostroenie, 280.
Alifanov, O. M., Artyukhin, E. A. (1988). Ekstremalnye metody resheniya nekorrektnykh zadach i ikh prilozheniya k obratnym zadacham teploobmena. Moscow: Nauka, 285.
Artyukhin, E. A. (1975). Opredelenie koeffitsienta temperaturoprovodnosti po dannym eksperimenta. Inzhenernofizicheskii zhurnal, 29 (1), 87–90.
Krukovskii, P. G. (1998). Obratnye zadachi teplomassoperenosa (obschii inzhenernyi podkhod). Kyiv: Institut tekhnicheskoi teplofiziki NAN Ukrainy, 218.
Alifanov, O. M., Vabischevich, P. N., Mikhailov, V. V., Nenarokomov, A. V., Polezhaev, Yu. V., Reznik, S. V. (2001). Osnovy identifikatsii i proektirovaniya teplovykh protsessov i sistem. Moscow: Logos, 400.
Kozdoba, L. A., Krukovskii P. G. (1982). Metody resheniya obratnykh zadach teploperenosa. Kyiv: Nauka i tekhnicheskii progress, 360.
Faddeev, D. K., Faddeeva, V. N. (1963). Vychislitelnye metody lineinoi algebry. Vol. 960. Fizmatgiz.
Krukovskii, P. G. (1994). Universalnyi programmnometodicheskii podkhod k resheniyu obratnykh zadach teplomassoperenosa (programma FRIEND). Identifikatsiya dinamicheskikh sistem i obratnye zadachi. Saint Petersburg, 1.
Kozdoba, L. A. (1992). Vychislitelnaya teplofizika. Kyiv: Naukova dumka, 224.
Bek, Dzh., Blakuell, B. (1989). Nekorrektnye obratnye zadachi teploprovodnosti. Moscow: Mir, 312.
Berkovskii, B. M., Nogotov, E. F. (1976). Raznostnye metody issledovaniya zadach teploobmena. Minsk: Nauka i tekhnika, 141.
Krukovskii, P. G. (2003). Raschetnoeksperimentalnyi podkhod k analizu protsessov teplomassoobmena (metodologiya i primery primeneniya). Promyshlennaya teplotekhnika, 25 (4), 396–398.
DSTU, B. (2007). 1.114: 2007 (EN 13654: 1999, NEQ). Kolony. Metod vyprobuvannia na vohnestiikist (2007). Nakaz Ministerstva rehionalnoho rozvytku ta budivnytstva Ukrainy No. 63. 22.06.2007. Kyiv: Minrehionbud Ukrainy.
DSTU B V.1.1–4–98*. Zakhyst vid pozhezhi. Budivelni konstruktsii. Metody vyprobuvan na vohnestiikist. Zahalni vymohy. Chynnyi vid 19981028. (2005). Vyd. Derzhbud Ukrainy, 18.
Kovalov, A. І., Otrosh, Yu. A., Kosse, A. G., Chernenko, O. M. (2020). Dependence of the accuracy of determining the thermophysical characteristics of fire retardant coatings on the parameters of the model. Problemy pozharnoi bezopasnosti, 48, 63–70.
Kovalov, A., Otrosh, Y., Surianinov, M., Kovalevska, T. (2019). Experimental and Computer Researches of Ferroconcrete Floor Slabs at HighTemperature Influences. Materials Science Forum, 968, 361–367. doi: http://doi.org/10.4028/www.scientific.net/msf.968.361
Kovalev, A. I. (2012). Vliyanie pogreshnostei v izmerenii temperatur na tochnost opredeleniya teplofizicheskikh kharakteristik pokrytii monolitnykh zhelezobetonnykh perekritii. Pozhezhna bezpeka: teorіya і praktika, 12, 41–45.
Kovalov, A. I., Otrosh, Y. A., Kovalevska, T. M., Safronov, S. O. (2019). Methodology for assessment of the fireresistant quality of reinforcedconcrete floors protected by fireretardant coatings. IOP Conference Series: Materials Science and Engineering, 708 (1), 012058. doi: http://doi.org/10.1088/1757899x/708/1/012058
Kovalov, A. I. (2017). The Influence of Random Errors in the Measurement of Temperatures on the Inaccuracy of Determination of Thermophysical Characteristics of Coatings of Reinforced Concrete Floors. Problemi pozharnoi bezopasnosty, 41, 87–91.
Kovalev, A. I., Krukovskii, P. G., Abramov, A. A. (2012). Analiz vliyaniya oshibok izmereniya temperatur na pogreshnost opredeleniya teplofizicheskikh i ognezaschitnykh kharakteristik pokrytii zhelezobetonnykh perekritii. Pozhezhna bezpeka: teorіya і praktika, 10, 66–72.
Kovalov, A. I., Kachkar, Ye. V., Zobenko, N. V., Tyshchenko, O. M. (2014). Osoblyvosti zastosuvannia pokryttiv metalevykh konstruktsii pry riznykh temperaturnykh rezhymakh pozhezhi. Pozhezhna bezpeka: teoriia i praktyka, 16, 135–139.
Kovalov, A. I., Otrosh, Yu. A., Tomenko, V. I., Danilin, O. M., Bezuhla, Yu. S., Karpets, K. M. (2020). Estimation of the fireprotective capacity of reactive coatings of steel structures. Problemy nadzvychainykh sytuatsii, 2 (32), 44–55.
Kovalev, A. I., Dashkovskii, V. Iu. (2014). Research of the Fireproof Capability of “Amotherm Steel Wb” Coating for Metal Constructions Protection Using an ExperimentCalculation Method. Bezpieczeństwo i Technika Pożarnicza, 35, 107–113. doi: http://doi.org/10.12845/bitp.35.3.2014.9
Kovalev, A. I., Zobenko, N. V. (2016). Testing the Accuracy of Designating the Parameters of Intumescent Coatings of Metal Constructions. Bezpieczeństwo i Technika Pożarnicza, 43, 45–50. doi: http://doi.org/10.12845/bitp.43.3.2016.4
Kovalov, A. I., Zobenko, N. V. (2016). Preliminary assessment technique of coating flame retardant capacity for steel structures under hydrocarbon firetemperature conditions. Naukovyi visnyk: tsyvilnyi zakhyst ta pozhezhna bezpeka, 1, 59–65.
Kovalov, A., Slovinskyi, V., Udianskyi, M., Ponomarenko, I., Anszczak, M. (2020). Research of Fireproof Capability of Coating for Metal Constructions Using CalculationExperimental Method. Materials Science Forum, 1006, 3–10. doi: http://doi.org/10.4028/www.scientific.net/msf.1006.3
Kovalov, A. I., Zobenko, N. V., Otrosh, Yu. A., Khmyrov, I. M., Danilin, O. M. (2018). Accuracy of determination of parameters of coatings of steel structures under conditions of the temperature regime of a hydrocarbon fire. Problemi pozharnoi bezopasnosty, 43, 73–79.
Kovalov, A. I., Vedula, S. A., Hrushovinchuk, O. V. (2017). Peculiarities and problems of determination of the predicted durability term of steel constructions coatings. Visnyk Pryazovskoho derzhavnoho tekhnichnoho universytetu. Seriia: Tekhnichni nauky, 34, 232–238.
Nuianzin, V. M., Kovalov, A. I., Vedula, S. A. (2016). Experimental researchesof influence of climatic factors on flame retardant ability of coatings for steel structures. Visnyk KrNU imeni Mykhaila Ostrohradskoho, 5, 70–75.
Kovalov, A. I., Otrosh, Yu. A., Danilin, O. M., Aleksieieva, O. S., Khmyrov, I. M. (2018). Method of assessment of fire fighting safety coatings of steel constructions under the influence of climatic factors. Problemi pozharnoi bezopasnosty, 44, 49–56.
Kovalov, A. I., Otrosh, Y. A., Vedula, S., Danilin, O. M., Kovalevska, T. M. (2019). Parameters of fireretardant coatings of steel constructions under the influence of climatic factors. Naukovyi Visnyk Natsionalnoho Hirnychoho Universytetu, 3, 46–53. doi: http://doi.org/10.29202/nvngu/20193/9
DSTUN B EN 199312:2010 “Yevrokod 3. Proektuvannia stalevykh konstruktsii. Chastyna 12. Zahalni polozhennia. Rozrakhunok konstruktsii na vohnestiikist (EN 199312:2005,IDT)” (2010). Available at: http://online.budstandart.com/ua/catalog/docpage?id_doc=26637
DBN V.1.17:2016 Pozhezhna bezpeka ob`yektiv budivnytstva. Zahalni vymohy. Chynnyi vid 20170601 (2017). Vyd. Ukrainskyi naukovodoslidnyi instytut tsyvilnoho zakhystu UkrNDITsZ, 35.
Kovalov, A., Otrosh, Y., Chernenko, O., Zhuravskij, M., Anszczak, M. (2021). Modeling of NonStationary Heating of Steel Plates with FireProtective Coatings in Ansys under the Conditions of Hydrocarbon Fire Temperature Mode. Materials Science Forum, 1038, 514–523. doi: http://doi.org/10.4028/www.scientific.net/msf.1038.514
Tsoi, A. A., Demekhin, F. V. (2015). Testing of fire resistant materials in the conditions of the hydrocarbon temperat ure mode. Nauchnoanaliticheskii zhurnal Vestnik SanktPeterburgskogo universiteta Gosudarstvennoi protivopozharnoi sluzhby MCHS Rossii, 4, 20–24.
Kovalov, A. I., Kachkar, Ye. V., Zobenko, N. V., Dolishnii, Yu. V. (2014). Eksperymentalne doslidzhennia vohnezakhysnoi zdatnosti pokryttia Amotherm Steel Wb pry temperaturnomu rezhymovi vuhlevodnevoi pozhezhi. Pozhezhna bezpeka: teoriia i praktyka, 17, 53–60.
Kovalov, A. I., Slovinskyi, V. K. (2019). Research of fireproof capabilityofcoating for metal constructions using experiment and calculationmethod. Nadzvychaini sytuatsii: poperedzhennia ta likvidatsiia, 3 (2), 37–47.
Kovalev, A. I., Zobenko, N. V., Oliinik, I. Ya. (2017). Opredelenie kharakteristiki ognezaschitnoi sposobnosti pokritii metallicheskikh konstruktsii pri ikh ispitaniyakh v usloviyakh temperaturnogo rezhima uglevodorodnogo pozhara. New technologies and achievements in metallurgy, material engineering, production engineering and physics.
Kovalov, A. I., Otrosh, Yu. A., Tomenko, V. I. (2020). The modelling of the thermal state of the steel constructions under the hydrocarbon fire temperature mode. Problemy nadzvychainykh sytuatsii, 31, 187–197.
Kovalov, A. I. (2017). Doslidzhennia tochnosti vyznachennia parametriv pokryttiv dlia vohnezakhystu stalevykh konstruktsii. Promyslove budivnytstvo ta inzhenerni sporudy, 4, 11–15.
Vasilchenko, A., Otrosh, Y., Adamenko, N., Doronin, E., Kovalov, A. (2018). Feature of fire resistance calculation of steel structures with intumescent coating. MATEC Web of Conferences, 230, 02036. doi: http://doi.org/10.1051/matecconf/201823002036
Pospelov, B., Rybka, E., Meleshchenko, R., Krainiukov, O., Biryukov, I., Butenko, T. et. al. (2021). Shortterm fire forecast based on air state gain recurrence and zeroorder brown model. Eastern-European Journal of Enterprise Technologies, 3 (10 (111)), 27–33. doi: http://doi.org/10.15587/17294061.2021.233606
Guzii, S. G., Otrosh, Y., Guzii, O., Kovalov, A., Sotiriadis, K. (2021). Determination of the FireRetardant Efficiency of Magnesite Thermal Insulating Materials to Protect Metal Structures from Fire. Materials Science Forum, 1038, 524–530. doi: http://doi.org/10.4028/www.scientific.net/msf.1038.524
Kovalev, A. I. (2012). Vliyanie pogreshnostei v izmerenii temperatur na tochnost opredeleniya teplofizicheskikh kharakteristik pokrytii monolitnykh zhelezobetonnykh perekrytii. Pozhezhna bezpeka: teorіya і praktika, 12, 41–45.
Kovalev, A. I. (2007). K probleme otsenki adgezii ognezaschitnykh pokrytii Naukovii vіsnik budіvnitstva, 41, 273–275.
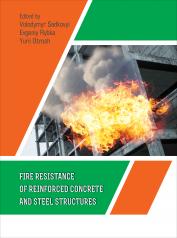
Downloads
Published
Categories
License

This work is licensed under a Creative Commons Attribution-NonCommercial-NoDerivatives 4.0 International License.